Difference between revisions of "Acetaminophen Manuscript"
(6 intermediate revisions by the same user not shown) | |||
Line 16: | Line 16: | ||
:Acetaminophen (Paracetamol, 4'-Hydroxyacetanilide, 4-Acetamidophenol, or APAP) is one of the most widely used analgesics and antipyretics and can be purchased over the counter. Moreover, APAP overdose remains to be a large problem in the United States and is responsible for a majority of acute liver failures annually (1,2). The quantitative difference between a safe dose and a toxic dose is narrow, and varies by individual based on environmental, genetic, and metabolic factors. Hence, it is deceptively easy to take too much APAP and cause a trip to the emergency room, permanent liver damage, or even death. | :Acetaminophen (Paracetamol, 4'-Hydroxyacetanilide, 4-Acetamidophenol, or APAP) is one of the most widely used analgesics and antipyretics and can be purchased over the counter. Moreover, APAP overdose remains to be a large problem in the United States and is responsible for a majority of acute liver failures annually (1,2). The quantitative difference between a safe dose and a toxic dose is narrow, and varies by individual based on environmental, genetic, and metabolic factors. Hence, it is deceptively easy to take too much APAP and cause a trip to the emergency room, permanent liver damage, or even death. | ||
+ | |||
:While APAP itself is harmless, APAP metabolic pathways have been investigated by numerous studies which allude to the reactive metabolites of APAP being responsible for liver necrosis. The formation of reactive metabolites coupled with glutathione depletion and alkylation of mitochondrial proteins are suggested to be the critical initiating events for APAP induced hepatotoxicity by way of causing mitochondrial permeability transition (MPT), which is an increase in permeability of the inner membrane that may lead to apoptosis or necrosis of liver cells (3). Reactive metabolites of APAP form via oxidation of APAP in the liver, which occurs after other nontoxic metabolic pathways such as glucuronidation and sulfation have been saturated. | :While APAP itself is harmless, APAP metabolic pathways have been investigated by numerous studies which allude to the reactive metabolites of APAP being responsible for liver necrosis. The formation of reactive metabolites coupled with glutathione depletion and alkylation of mitochondrial proteins are suggested to be the critical initiating events for APAP induced hepatotoxicity by way of causing mitochondrial permeability transition (MPT), which is an increase in permeability of the inner membrane that may lead to apoptosis or necrosis of liver cells (3). Reactive metabolites of APAP form via oxidation of APAP in the liver, which occurs after other nontoxic metabolic pathways such as glucuronidation and sulfation have been saturated. | ||
+ | |||
:APAP may undergo a one electron oxidation to yield the APAP phenoxyl radical (N-acetyl-p-benzosemiquinone imine; NAPSQI) or a direct two electron oxidation to produce N-acetyl-p-benzoquinone imine (NAPQI) via Cytochrome P450 enzymes (CYPs) (4). The result leads to hepatotoxicity after intracellular supplies of glutathione have been used up. The commonly accepted mechanism of oxidative metabolite formation during APAP overdose revolves around the direct two electron oxidation of APAP into NAPQI (5, 6). NAPQI is then purported to be reduced and detoxified via glutathione (GSH) conjugation through glutathione transferase, leading to cysteine and mercapturic acid metabolites to be formed (7). The formation of NAPQI has been indirectly identified as a metabolite of APAP oxidation within the liver through its conjugation with glutathione (6). The uncertainty associated with this indirect metabolic identification raises hesitance to NAPQI being the only actor during APAP overdose. Moreover, the oxidation of APAP by one electron to form NAPSQI may be independently reduced by GSH, reforming APAP and forming glutathione disulfide (GSSG) (8). Under scenarios of depleted glutathione reserves, this pathway may yield a different set of metabolites, which could play bioactive roles responsible for the MPT and resulting hepatotoxicity observed following APAP overdose. | :APAP may undergo a one electron oxidation to yield the APAP phenoxyl radical (N-acetyl-p-benzosemiquinone imine; NAPSQI) or a direct two electron oxidation to produce N-acetyl-p-benzoquinone imine (NAPQI) via Cytochrome P450 enzymes (CYPs) (4). The result leads to hepatotoxicity after intracellular supplies of glutathione have been used up. The commonly accepted mechanism of oxidative metabolite formation during APAP overdose revolves around the direct two electron oxidation of APAP into NAPQI (5, 6). NAPQI is then purported to be reduced and detoxified via glutathione (GSH) conjugation through glutathione transferase, leading to cysteine and mercapturic acid metabolites to be formed (7). The formation of NAPQI has been indirectly identified as a metabolite of APAP oxidation within the liver through its conjugation with glutathione (6). The uncertainty associated with this indirect metabolic identification raises hesitance to NAPQI being the only actor during APAP overdose. Moreover, the oxidation of APAP by one electron to form NAPSQI may be independently reduced by GSH, reforming APAP and forming glutathione disulfide (GSSG) (8). Under scenarios of depleted glutathione reserves, this pathway may yield a different set of metabolites, which could play bioactive roles responsible for the MPT and resulting hepatotoxicity observed following APAP overdose. | ||
+ | |||
:Possible metabolites associated with a one electron oxidation of APAP in the liver have been identified using model systems such as enzymatic or electrochemical methods of oxidation in place of liver microsomes. Following a one electron oxidation by a model enzyme, Horseradish peroxidase (HRP), studies have been done to characterize the APAP radical intermediate in solution through electron spin resonance (ESR) and the potential for this radical to from a different set of metabolites that are not NAPQI (9,10). Characterization of the postulated metabolites allowed for the identification of a set of APAP polymers consistent with a mechanism of radical polymerization (11). Deeper investigation into such metabolites and their radical intermediates could shed light on the controversial topic of how APAP induced liver necrosis disseminates. Considering that, the purpose of this work is to revisit the oxidation of APAP and its downstream oxidation products in order to elucidate a better understanding of the chemistry occurring during this reaction. | :Possible metabolites associated with a one electron oxidation of APAP in the liver have been identified using model systems such as enzymatic or electrochemical methods of oxidation in place of liver microsomes. Following a one electron oxidation by a model enzyme, Horseradish peroxidase (HRP), studies have been done to characterize the APAP radical intermediate in solution through electron spin resonance (ESR) and the potential for this radical to from a different set of metabolites that are not NAPQI (9,10). Characterization of the postulated metabolites allowed for the identification of a set of APAP polymers consistent with a mechanism of radical polymerization (11). Deeper investigation into such metabolites and their radical intermediates could shed light on the controversial topic of how APAP induced liver necrosis disseminates. Considering that, the purpose of this work is to revisit the oxidation of APAP and its downstream oxidation products in order to elucidate a better understanding of the chemistry occurring during this reaction. | ||
Line 23: | Line 26: | ||
:Procedures for experimental methods should be described in sufficient detail to enable other investigators to repeat the experiments. Names of product manufacturers (with city, state address, catalog number) should be included if alternate sources are deemed unsatisfactory or if the product is of limited availability. Novel experimental procedures should be described in detail, but previously published procedures should be referred to by literature citation of the original detailed explanation, and should include description of any modifications. | :Procedures for experimental methods should be described in sufficient detail to enable other investigators to repeat the experiments. Names of product manufacturers (with city, state address, catalog number) should be included if alternate sources are deemed unsatisfactory or if the product is of limited availability. Novel experimental procedures should be described in detail, but previously published procedures should be referred to by literature citation of the original detailed explanation, and should include description of any modifications. | ||
+ | :Acetaminophen, H2O2 (30%), dioxane, methanol, buffer tablets, deuterated dimethyl sulfoxide, and Horseradish peroxidase (Type II) were purchased from Sigma Aldrich. | ||
+ | |||
+ | :''Acetaminophen Oxidation'' | ||
+ | :Acetaminophen oxidations were carried out with 50mM acetaminophen, 0-50mM H2O2, 0-9.7nm HRP, in 25% dioxane to aqueous saline buffer (pH 9.2). Samples were equilibrated at room temperature and then initiated by the addition of H2O2. Reactions were allowed to go until completion. Acetaminophen oxidation products were then analyzed and isolated. | ||
− | : | + | :''Liquid Chromatography'' |
+ | :The analytical HPLC system that was used for analysis was an Agilent 1100 HPLC paired with a diode array detector (190 nm - 850 nm). Both a reverse phase C18 column and a normal phase amine column were utilized for separation. A binary solvent system with a flow rate of 1.0 mL/minute containing Solvent A, 0.1% trifluoroacetic acid, and Solvent B, 100% acetonitrile, allowed for the separation and quantification of acetaminophen metabolites following oxidation. For reverse phase HPLC, Solvent A was held at 100% for the first minute of the run, followed by a linear transition to 100% solvent B until minute 15 at which Solvent B was held constant for 3 more minutes. For normal phase HPLC, Solvent B was held at 100% for the first minute of the run, followed by a linear transition to 50% solvent A until minute 15 at which Solvent A was held constant for 3. BOth methods gave a total run time of 18 minutes. | ||
+ | :The automated flash chromatography system used to purify Acetaminophen metabolites for characterization was a Teledyne Isco CombiFlash Rf 200i system. Purification was carried out via a 5.5mg C18 gold column with a flow rate of 18 mL/minute. Solvent A was held constant at 100% for 1 column volume, followed by a linear transition to 75% Acetonitrile over the course of 29 column additional column volumes. Acetaminophen metabolite fractions were collected and the solvent mixtures were removed using a rotary evaporator. | ||
− | '' | + | :''Mass Spectrometry'' |
− | : | + | :Electrospray ionization was utilized with a quadruple time-of-flight tandem mass analyzer for the analysis of purified acetaminophen metabolites solubilized in methanol. Samples were introduced to the mass spectrometer using direct injection. |
+ | :''Nuclear Magnetic Resonance Spectroscopy'' | ||
+ | :H-NMR spectra were obtained using a 400 MHz Bruker NMR spectrometer. Spectra were obtained at room temperature with samples solubilized in deuterated dimethyl sulfoxide. | ||
− | '' | + | :''Electron Spin Resonance'' |
− | : | + | :ESR spectra were measured at room temperature using an Bruker EMX EPR Spectrometer with an SHQ resonator fitted with a 10 mm flat cell containing an immobilized enzyme system. The immobilized enzyme system was made using 1 mL of Affi-Gel® 10 beads and 10 mg HRP and inserted into the flat cell. Computer simulation of the experimental ESR spectra were carried out using WINSIM. |
+ | :''Computational Work'' | ||
+ | :WebMO and Gaussian were utilized to gather electron spin density information of the acetaminophen radical intermediate. Molecular orbital calculations were gathered using B3LYP/EPR-II as a basis set. | ||
− | :The | + | ==Results== |
+ | '':The results should be presented concisely. Tables and figures should be designed to maximize the presentation and comprehension of the experimental data. The same data should not be presented in more than one figure or in both a figure and a table. Detailed interpretation of results should be reserved for the discussion section of an Article.'' | ||
+ | [[File:APAP_ESR_spectra(2).png|thumb|left|350px|Figure 1: The data was ccollected according to ESR methods described above while perating at 9.8 GHz, 20 mW microwave power, 60 Gauss sweep width, and 0.5 Gpp modulation amplitude (100KHz). A 2mM APAP, 1mM H2O2, phosphate-citrate buffer (pH 5) solution was flowed through the IE system in order to generate the experimental spectra.]]Oxidation of APAP through the IE-ESR system allowed for the detection of a free radical (Figure 1). Low resolution modulation (0.5 G) allowed for a high resolution ESR spectrum with high signal to noise as illustrated in spectrum A. The assignment of hyperfine coupling constants was done using computer software to give a simulation of the full experimental spectrum in spectrum B. Mathematical removal of the signal associated with species 2 yielded the simulated spectrum C while the removal of the species 1 signal resulted in spectrum D. The assignment of the hyperfine coupling values of spectrum B (APAP radical) is summarized in Table # due to the removal of the signal associated with further polymerized APAP radical background noise being mathematically removed. The formation of the radical was dependent upon the presence of APAP, HRP, and H2O2. | ||
+ | [[File:pH9.png|350px|thumb|right|Figure 2: Reversed-phase HPLC separation of acetaminophen oxidation products as catalyzed by HRP with varying concentrations of H2O2 and constant acetaminophen levels. The reaction progress was monitored by a diode array detector (270 nm).]] | ||
− | |||
− | |||
+ | In order to better understand the oxidation of APAP, both in vitro “beaker” oxidations by HRP at varying pHs (pH 5.0, 7.4, and 9.2) and electrochemical oxidations were carried out. The result was the same product formation whether considering electrochemical or enzymatic methods of oxidation. Figure 2 depicts the HRP mediated oxidation of APAP to completion in 25% dioxane - pH 9.2 buffer since clearly visualizable product formation was maximized under those conditions. Dioxane was utilized as a solvent since it could both solubilize the water insoluble APAP oxidation products and retain the functionality of HRP. | ||
+ | [[File:Polymer_Oxidation.png|350px|thumb|right|Figure 3: Isolated metabolites were oxidized using the previously described H2O2/HRP beaker oxidation system. 1 mM compound A (red) and 1 mM compound B (blue) were oxidized with the addition of 0.5 mM H2O2. The reactions were carried out in a 50% dioxane - pH 5.0 buffer. The bulk APAP/H2O2/HRP reaction in black serves as a product standard.]] | ||
+ | [[File:qTOF-MS_table(5).png|250px|thumb|left|Table 1: ESI-MS/MS analysis of acetaminophen metabolites solubilized in methanol was gathered. The data represents a partial set of molecular ions as a result of the flash purified metabolites isolated from the APAP/H2O2/HRP oxidation reaction. ESI-MS data are m/z + 1 and contain relative abundance of each molecular ion.]] | ||
− | |||
− | |||
+ | The oxidation of APAP by HRP with increasing H2O2 concentrations allowed for the formation of five clearly distinguished products and the disappearance of APAP as a result of the progression of the reaction (Figure 2). The products (A, B, C, D, and E) were then isolated, and subjected to mass spectral analysis using ESI-MS (Table 1). The soft ionization allowed for the parent ion of each product to be retained. The m/z ratio associated with compound A through E are consistent with product formation of conjugated APAP polymers given by the equation: Mass = (n x APAP) - (n x H+) with mass being the detected m/z ratio, n being the number of APAP molecules in the polymer, and H+ being the number of hydrogen atoms lost due to conjugation of APAP molecules with each other. | ||
− | |||
− | |||
− | |||
+ | Given that, compounds A and B are strongly suggested to be a dimer and trimer of APAP, respectively. Following the same reasoning, compounds C, D, and E are consistent with the formation of two APAP tetramers and a pentamer. Compounds A and B were further oxidized under the same conditions as APAP in order to visualize product outcome by way of their individual oxidation by HRP (Figure 3). The oxidation of compound A yielded a product that eluted off of the reverse phase column at the same time as compound C while the oxidation of compound B yielded a product that elutes at approximately the same time as compound E. | ||
− | |||
− | |||
− | + | Compound A, the APAP dimer (diAPAP) and earliest formed metabolite during APAP oxidation, was further investigated using H-NMR. The zoomed in aromatic regions are shown for both the experimentally purified APAP dimer as well as a purchased ortho-linked APAP dimer with the purpose of being used as a standard (Figure 4). The fact that compound A holds similarity too, but also unique splitting patterns from the ortho-linked APAP dimer standard indicates that the enzymatic oxidation of APAP yields a mixture of diAPAP isomers. This was further visulaized by through normal phase HPLC, in which poor but sufficient separation allowed for the visualization of two chemically unique absorbance peaks (Figure 6). The two geometric isomers were attributed to the prescence of an ortho-linked diAPAP and an ether-linked diAPAP. | |
− | |||
− | + | [[File:stacked_diapap_NMR.png|500px|thumb|left|Figure 4:]] [[File:diAPAP_Chromatography.png|350px|thumb|center| Figure 6: Compound A chromatography was attempted using both reverse phase HPLC (red) as was done prior and normal phase HPLC (black).]] | |
− | |||
− | :[[File: | + | [[File:diAPAP_ESR.png|400px|thumb|left|Figure 8: ESR data of compound A (diAPAP): low resolution solo oxidation of 1 mM diAPAP and 0.5 mM H2O2, low resolution oxidation of diAPAP with the addition of 0.2 mM APAP, high resolution oxidation of diAPAP with fractional APAP, and a simulation of the high resolution spectra.]] [[File:diAPAP_IE_ESR.png|300px|thumb|right|Figure 9: diAPAP prior to reaction can be seen in red while product outcomes can be seen in blue at different flowrates through the IE method. On top, diAPAP is oxidized by this system alone. On the bottom, diAPAP oxidation is joined with fractional levels of APAP.]] |
+ | Oxidation of diAPAP through the IE-ESR system allowed for the detection of a radical intermediate as illustrated at the top of Figure 7. The signal associated with the oxidation of diAPAP gave a broadband polymeric signal. Spiking the solution with a fractional amount of APAP allowed for a larger signal and for unique character to develop, which is shown just below the top spectra. Switching from a modulation amplitude of 1.0 G to 0.5 G gave high resolution as seen in black. Hyperfine coupling constants were then assigned to this highly resolved signal via the use of computer software. The simulation can be visualized in red just below the experimentally collected data. The determined hyperfine coupling constants of diAPAP were exactly half that of the APAP. The ortho protons, aH, and amide nitrogens, aN, had simulated constants of 2.53 G and 0.88 G compared to APAP’s simulated constants of 5.19 G and 1.68 G, respectively. | ||
− | + | Given that, spiking the diAPAP sample with fractional APAP levels resulted in an increase in the radical concentration of diAPAP. The oxidation products associated with the solo diAPAP oxidation and the APAP spiked diAPAP oxidation through the IE-ESR system is portrayed in Figure 8. diAPAP oxidation resulting in the broadband ESR spectra of Figure 8 results in product formation of compound C, an acetaminophen tetramer. However, when spiked with fractional APAP, the main product outcome of diAPAP oxidation was compound B, an acetaminophen trimer, followed by compound C and further conjugated polymers. | |
− | + | ==Discussion== | |
− | + | :''The purpose of the discussion is to interpret the results and to relate them to existing knowledge in the field in as clear and brief a fashion as possible. Information given elsewhere in the manuscript should not be repeated in the discussion. Extensive reviews of the literature should be avoided.'' | |
− | :The | ||
+ | Clearly, APAP oxidation is not so simple so as to only lead to the formation of one, clear cut, metabolite during oxidation in times of overdose. While NAPQI may be a product of APAP oxidation in the liver, products of a one electron oxidation cascade cannot be written out and remain prevalent. Five major APAP oxidation products were shown to be formed after modeling the CYP mediated reaction with HRP, a classic peroxidase. CYPs have been shown to catalyze one electron oxidations of phenolic compounds in environments of oxidative stress, which is certaintly the case with APAP overdose once glutathione levels have been depleted (4). Perhaps the metabolism of APAP after gluthione depletion favors the mechanism of radical polymerization as opposed to the net two electron oxidation of APAP into NAPQI. Considering the former could clear up discrepencies when considering the mystery of how APAP induced liver necrosis disseminates. For instance, while polyphenolic compounds are generally seen as safe supplements, high concentration seem to function antagonistically and were purported to lead to increases in DNA damage (12). If APAP is oxidized via a mechanism of one electron oxidation that yields polymers, then the concentration of polyphenols being formed would be classified as very high. This could elucidate the mechanism of mitochondrial dysfunction often associated with APAP overdose in the liver. If mitochondrial DNA is being damaged, then the kill switches, necrosis or apoptosis, could be signaled for in hepatocytes, thus bringing about APAP induced hepatotoxicity. | ||
− | + | According to the ESR data collected, APAP appears to be most likely to react at the ortho carbon position to form an ortho-linked dimer (diAPAP). This is supported by previous APAP dimer identification performed by Potter in 1985; however, there was an additional geometric isomer of the APAP dimer that Potter did not characterize (11). The additional APAP dimer was found to be an ether-linked diAPAP molecule. Additionally, the diAPAP ESR indicates the mechanism of APAP oxidation by HRP is APAP mediated or APAP induced. In other words, APAP drives the reaction forward. To clarify, diAPAP does function as a substrate for the peroxidase, but does not yield large product fromation when compared to spiking the solution with low levels of APAP. Introducing APAP to the oxidation of diAPAP yielded far greater product outcomes as well as the depletion of diAPAP. It can be reasoned that APAP may be oxidized into a radical intermediate, which then directly adducts to diAPAP to form immediate APAP trimer or stimulates diAPAP oxidation by stealing an electron from diAPAP to generate the diAPAP radical and become reduced back to APAP. | |
− | |||
+ | ==References== | ||
+ | 1. Lee, W. M. (2013) [[Media:Lee; Drug Induced Liver Failure.pdf|'''Drug-induced acute liver failure''']]. ''Clin Liver Dis.'' 17, 575-586. | ||
− | ''' | + | 2. Bernal, W., Lee, W. M., Wendon, J., Larsen, F. S., and Williams, R. (2015) [[Media:A curable disease.pdf|'''Acute liver failure: A curable disease by 2024?''']]. ''J. Hepatol.'' 62, 112-120. |
− | |||
− | |||
− | |||
− | |||
− | |||
− | |||
+ | 3. Hinson, J. A., Reid, A. B., McCullough, S. S., and James, L. P. (2004) [[Media:MPT.pdf|Acetaminophen‐Induced Hepatotoxicity:'''Role of Metabolic Activation, Reactive Oxygen/Nitrogen Species, and Mitochondrial Permeability Transition''']]. ''Drug Metabolism Reviews'' 36(3-4), 805–822. | ||
− | ''' | + | 4. Potter, D. W. and Hinson, J. A. (1987) [[Media:CYP versus HRP.pdf|'''Mechanisms of acetaminophen oxidation to N-acetyl-P-benzoquinone imine by horseradish peroxidase and cytochrome P-450''']]. ''J Biol Chem.'' 262, 966–973. |
− | |||
− | |||
− | |||
+ | 5. Gillette, J. R., Nelson, S. D., Mulder, G. J., Jollow, D. J., Mitchell, J. R., Pohl, L. R., and Hinson, J. A. (1981) '''Formation of chemically reactive metabolites of phenacetin and acetaminophen.''' ''Adv Exp Med Biol.'' 136, 931–950. | ||
− | ''' | + | 6. Dahlin, D. C., Miwa, G. T., Lu, A. Y., and Nelson, S. D. (1984) [[Media:NAPQI discovery.pdf|'''N-acetyl-p-benzoquinone imine: a cytochrome P-450-mediated oxidation product of acetaminophen''']]. ''Proc. Natl. Acad. Sci. U. S. A.'' 81, 1327-1331. |
− | |||
+ | 7. Mitchell, J. R., Jollow, D. J., Potter, W. Z., Gillette, J. R., and Brodie, B. B. (1973b) '''Acetaminophen-induced hepatic necrosis. IV. Protective role of glutathione'''. ''J Pharmacol Exp Ther.'' 187, 211–217. | ||
− | ''' | + | 8. Ross, D., Norbeck, K., and Moldeus, P. (1985) [[Media:Glutathione radical fate.pdf|'''The Generation and Subsequent Fate of Glutathionyl Radicals in Biological Systems''']]. ''Journal of Biological Chemistry'' 260(28), 15028-15032. |
− | |||
+ | 9. West, P. R., Harman, L. S., Josephy, P. D., and Mason, R. P.(1984) [[Media:West 1984.pdf|'''Acetaminophen: enzymatic formation of a transient phenoxyl free radical''']]. ''Journal of Biochemical Pharmacology'' 33(18), 2933-2936. | ||
− | ''' | + | 10. Fischer, V., West, P. R., Harman, L. S., and Mason, R. P. (1985) [[Media:second APAP ESR.pdf|'''Free-Radical Metabolites of Acetaminophen and a Dimethylated Derivative''']]. ''Environmental Health Perspectives''64, 127-137. |
− | |||
− | ''' | + | 11. Potter, D. W., Miller, D. W., and Hinson, J. A. (1985) [[Media:J._Biol._Chem.-1985-Potter-12174-80.pdf|'''Identification of Acetaminophen Polymerization Products Catalyzed by Horseradish Peroxidase.''']] ''J. Biol. Chem.'' 260(12), 174-180. |
− | |||
− | + | 12. Aqueta, A. and Collins, A. (2016) [[Media:nutrients.pdf|'''Polyphenols and DNA Damage: A Mixed Blessing''']]. ''Nutrients'' 8(785), 1-21. | |
− | : | ||
==Funding Information== | ==Funding Information== |
Latest revision as of 13:11, 7 July 2022
This page is being prepared to submit a manuscript for peer review and publication in the Journal of Chemical Research in Toxicology by Matthew Simonson. The goal of this work is to provide foundational evidence for a mechanism of hepatotoxicity caused by radical polymerization of Acetaminophen within the liver as opposed to soley a mechanism of two electron oxidation into NAPQI.
Title Page
- A brief and informative title (preferably fewer than 12 words) will aid in the classification and indexing of the paper. Do not use trade names of drugs, jargon, or abbreviations. Include keywords. List full names and institutional affiliations of all authors, and if differentiation is necessary, indicate the affiliations of each author by the superscript symbols †, ‡, §, ║, ┴, etc. These symbols should also be used to indicate author affiliations different from those stated on the title page and present address information. The author to whom correspondence should be addressed is indicated by an asterisk. It is implicit in listing a person as an author that this individual has agreed to appear as an author of the manuscript.
Table of Contents Graphic
- A Table of Contents (TOC) graphic is published with each manuscript. It is submitted for use in the table of contents and is also used for multiple purposes, including the document abstract and other situations where a representative graphic is required. Create an image that represents the work while adhering to size constraints. Keeping in mind that various devices may be involved, some of the best images are simple, relatively free of text and technical characters, and make use of color for visual impact. It is best to avoid complex structure schemes and small-sized details. The author must submit a graphic in the actual size to be used for the TOC that will fit in an area 8.47 cm by 4.76 cm (3.33 in. by 1.88 in.). Larger images will be reduced to fit within those dimensions. Type size of labels, formulas, or numbers within the graphic must be legible at the specified size. Tables or spectra are not acceptable. Place the TOC graphic after the title page and before the abstract page of the manuscript. All elements of the TOC graphic must be (1) entirely original and (2) created by one or more of the authors. Lastly, this graphic should have no legend.
Abstract
- An abstract should be included with all Articles, Communications, Chemical Profiles, Reviews, and Perspectives. For Articles, Communications, and Chemical Profiles, the abstract should briefly (300 word maximum) present, in one paragraph, the problem and experimental approach and state the findings and conclusions. For Reviews and Perspectives, the abstract should introduce the topic, summarize key points, and state the major conclusions. In all cases, the abstract should be self- explanatory and suitable for reproduction without rewriting. Footnotes or undefined abbreviations may not be used. Avoid the use of jargon, but include keywords relevant to the field to improve indexing and discoverability to potential readers. If a reference must be cited, complete publication data must be given.
- Acetaminophen (APAP) is a common analgesic and an active ingredient in many painkillers such as Tylenol and Percocet. Upon overdose, APAP can lead to toxicity in the liver, which accounts for a striking proportion of acute liver failures in the United States annually. Considering the potential for APAP induced hepatotoxicity, our research group has analyzed APAP and its tendency to oxidize into reactive metabolites through enzymatic methods of in vitro oxidation, which was visualized through HPLC pairing with a diode array detector. Characterization of these APAP oxidation products were carried out using electron spin resonance (ESR), electrospray ionization tandem mass spectrometry (ESI-MS/MS), and 400 MHz proton nuclear magnetic resonance (H-NMR). Our findings provide structural and mechanistic insight into the radical propagation of potentially deleterious APAP metabolites formed in the liver during times of APAP overdose.
Introduction
- The introduction should state the purpose of the investigation and its relation to other work in the field. Background material should be brief and relevant to the research described. Detailed or lengthy reviews of the literature should be avoided.
- Acetaminophen (Paracetamol, 4'-Hydroxyacetanilide, 4-Acetamidophenol, or APAP) is one of the most widely used analgesics and antipyretics and can be purchased over the counter. Moreover, APAP overdose remains to be a large problem in the United States and is responsible for a majority of acute liver failures annually (1,2). The quantitative difference between a safe dose and a toxic dose is narrow, and varies by individual based on environmental, genetic, and metabolic factors. Hence, it is deceptively easy to take too much APAP and cause a trip to the emergency room, permanent liver damage, or even death.
- While APAP itself is harmless, APAP metabolic pathways have been investigated by numerous studies which allude to the reactive metabolites of APAP being responsible for liver necrosis. The formation of reactive metabolites coupled with glutathione depletion and alkylation of mitochondrial proteins are suggested to be the critical initiating events for APAP induced hepatotoxicity by way of causing mitochondrial permeability transition (MPT), which is an increase in permeability of the inner membrane that may lead to apoptosis or necrosis of liver cells (3). Reactive metabolites of APAP form via oxidation of APAP in the liver, which occurs after other nontoxic metabolic pathways such as glucuronidation and sulfation have been saturated.
- APAP may undergo a one electron oxidation to yield the APAP phenoxyl radical (N-acetyl-p-benzosemiquinone imine; NAPSQI) or a direct two electron oxidation to produce N-acetyl-p-benzoquinone imine (NAPQI) via Cytochrome P450 enzymes (CYPs) (4). The result leads to hepatotoxicity after intracellular supplies of glutathione have been used up. The commonly accepted mechanism of oxidative metabolite formation during APAP overdose revolves around the direct two electron oxidation of APAP into NAPQI (5, 6). NAPQI is then purported to be reduced and detoxified via glutathione (GSH) conjugation through glutathione transferase, leading to cysteine and mercapturic acid metabolites to be formed (7). The formation of NAPQI has been indirectly identified as a metabolite of APAP oxidation within the liver through its conjugation with glutathione (6). The uncertainty associated with this indirect metabolic identification raises hesitance to NAPQI being the only actor during APAP overdose. Moreover, the oxidation of APAP by one electron to form NAPSQI may be independently reduced by GSH, reforming APAP and forming glutathione disulfide (GSSG) (8). Under scenarios of depleted glutathione reserves, this pathway may yield a different set of metabolites, which could play bioactive roles responsible for the MPT and resulting hepatotoxicity observed following APAP overdose.
- Possible metabolites associated with a one electron oxidation of APAP in the liver have been identified using model systems such as enzymatic or electrochemical methods of oxidation in place of liver microsomes. Following a one electron oxidation by a model enzyme, Horseradish peroxidase (HRP), studies have been done to characterize the APAP radical intermediate in solution through electron spin resonance (ESR) and the potential for this radical to from a different set of metabolites that are not NAPQI (9,10). Characterization of the postulated metabolites allowed for the identification of a set of APAP polymers consistent with a mechanism of radical polymerization (11). Deeper investigation into such metabolites and their radical intermediates could shed light on the controversial topic of how APAP induced liver necrosis disseminates. Considering that, the purpose of this work is to revisit the oxidation of APAP and its downstream oxidation products in order to elucidate a better understanding of the chemistry occurring during this reaction.
Experimental Procedures
- Procedures for experimental methods should be described in sufficient detail to enable other investigators to repeat the experiments. Names of product manufacturers (with city, state address, catalog number) should be included if alternate sources are deemed unsatisfactory or if the product is of limited availability. Novel experimental procedures should be described in detail, but previously published procedures should be referred to by literature citation of the original detailed explanation, and should include description of any modifications.
- Acetaminophen, H2O2 (30%), dioxane, methanol, buffer tablets, deuterated dimethyl sulfoxide, and Horseradish peroxidase (Type II) were purchased from Sigma Aldrich.
- Acetaminophen Oxidation
- Acetaminophen oxidations were carried out with 50mM acetaminophen, 0-50mM H2O2, 0-9.7nm HRP, in 25% dioxane to aqueous saline buffer (pH 9.2). Samples were equilibrated at room temperature and then initiated by the addition of H2O2. Reactions were allowed to go until completion. Acetaminophen oxidation products were then analyzed and isolated.
- Liquid Chromatography
- The analytical HPLC system that was used for analysis was an Agilent 1100 HPLC paired with a diode array detector (190 nm - 850 nm). Both a reverse phase C18 column and a normal phase amine column were utilized for separation. A binary solvent system with a flow rate of 1.0 mL/minute containing Solvent A, 0.1% trifluoroacetic acid, and Solvent B, 100% acetonitrile, allowed for the separation and quantification of acetaminophen metabolites following oxidation. For reverse phase HPLC, Solvent A was held at 100% for the first minute of the run, followed by a linear transition to 100% solvent B until minute 15 at which Solvent B was held constant for 3 more minutes. For normal phase HPLC, Solvent B was held at 100% for the first minute of the run, followed by a linear transition to 50% solvent A until minute 15 at which Solvent A was held constant for 3. BOth methods gave a total run time of 18 minutes.
- The automated flash chromatography system used to purify Acetaminophen metabolites for characterization was a Teledyne Isco CombiFlash Rf 200i system. Purification was carried out via a 5.5mg C18 gold column with a flow rate of 18 mL/minute. Solvent A was held constant at 100% for 1 column volume, followed by a linear transition to 75% Acetonitrile over the course of 29 column additional column volumes. Acetaminophen metabolite fractions were collected and the solvent mixtures were removed using a rotary evaporator.
- Mass Spectrometry
- Electrospray ionization was utilized with a quadruple time-of-flight tandem mass analyzer for the analysis of purified acetaminophen metabolites solubilized in methanol. Samples were introduced to the mass spectrometer using direct injection.
- Nuclear Magnetic Resonance Spectroscopy
- H-NMR spectra were obtained using a 400 MHz Bruker NMR spectrometer. Spectra were obtained at room temperature with samples solubilized in deuterated dimethyl sulfoxide.
- Electron Spin Resonance
- ESR spectra were measured at room temperature using an Bruker EMX EPR Spectrometer with an SHQ resonator fitted with a 10 mm flat cell containing an immobilized enzyme system. The immobilized enzyme system was made using 1 mL of Affi-Gel® 10 beads and 10 mg HRP and inserted into the flat cell. Computer simulation of the experimental ESR spectra were carried out using WINSIM.
- Computational Work
- WebMO and Gaussian were utilized to gather electron spin density information of the acetaminophen radical intermediate. Molecular orbital calculations were gathered using B3LYP/EPR-II as a basis set.
Results
:The results should be presented concisely. Tables and figures should be designed to maximize the presentation and comprehension of the experimental data. The same data should not be presented in more than one figure or in both a figure and a table. Detailed interpretation of results should be reserved for the discussion section of an Article.
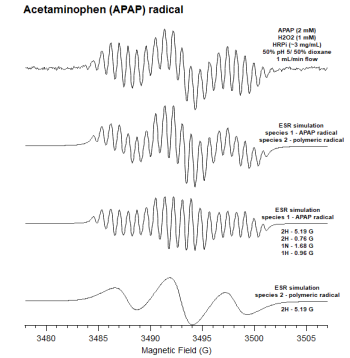
Oxidation of APAP through the IE-ESR system allowed for the detection of a free radical (Figure 1). Low resolution modulation (0.5 G) allowed for a high resolution ESR spectrum with high signal to noise as illustrated in spectrum A. The assignment of hyperfine coupling constants was done using computer software to give a simulation of the full experimental spectrum in spectrum B. Mathematical removal of the signal associated with species 2 yielded the simulated spectrum C while the removal of the species 1 signal resulted in spectrum D. The assignment of the hyperfine coupling values of spectrum B (APAP radical) is summarized in Table # due to the removal of the signal associated with further polymerized APAP radical background noise being mathematically removed. The formation of the radical was dependent upon the presence of APAP, HRP, and H2O2.
In order to better understand the oxidation of APAP, both in vitro “beaker” oxidations by HRP at varying pHs (pH 5.0, 7.4, and 9.2) and electrochemical oxidations were carried out. The result was the same product formation whether considering electrochemical or enzymatic methods of oxidation. Figure 2 depicts the HRP mediated oxidation of APAP to completion in 25% dioxane - pH 9.2 buffer since clearly visualizable product formation was maximized under those conditions. Dioxane was utilized as a solvent since it could both solubilize the water insoluble APAP oxidation products and retain the functionality of HRP.

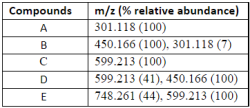
The oxidation of APAP by HRP with increasing H2O2 concentrations allowed for the formation of five clearly distinguished products and the disappearance of APAP as a result of the progression of the reaction (Figure 2). The products (A, B, C, D, and E) were then isolated, and subjected to mass spectral analysis using ESI-MS (Table 1). The soft ionization allowed for the parent ion of each product to be retained. The m/z ratio associated with compound A through E are consistent with product formation of conjugated APAP polymers given by the equation: Mass = (n x APAP) - (n x H+) with mass being the detected m/z ratio, n being the number of APAP molecules in the polymer, and H+ being the number of hydrogen atoms lost due to conjugation of APAP molecules with each other.
Given that, compounds A and B are strongly suggested to be a dimer and trimer of APAP, respectively. Following the same reasoning, compounds C, D, and E are consistent with the formation of two APAP tetramers and a pentamer. Compounds A and B were further oxidized under the same conditions as APAP in order to visualize product outcome by way of their individual oxidation by HRP (Figure 3). The oxidation of compound A yielded a product that eluted off of the reverse phase column at the same time as compound C while the oxidation of compound B yielded a product that elutes at approximately the same time as compound E.
Compound A, the APAP dimer (diAPAP) and earliest formed metabolite during APAP oxidation, was further investigated using H-NMR. The zoomed in aromatic regions are shown for both the experimentally purified APAP dimer as well as a purchased ortho-linked APAP dimer with the purpose of being used as a standard (Figure 4). The fact that compound A holds similarity too, but also unique splitting patterns from the ortho-linked APAP dimer standard indicates that the enzymatic oxidation of APAP yields a mixture of diAPAP isomers. This was further visulaized by through normal phase HPLC, in which poor but sufficient separation allowed for the visualization of two chemically unique absorbance peaks (Figure 6). The two geometric isomers were attributed to the prescence of an ortho-linked diAPAP and an ether-linked diAPAP.
Oxidation of diAPAP through the IE-ESR system allowed for the detection of a radical intermediate as illustrated at the top of Figure 7. The signal associated with the oxidation of diAPAP gave a broadband polymeric signal. Spiking the solution with a fractional amount of APAP allowed for a larger signal and for unique character to develop, which is shown just below the top spectra. Switching from a modulation amplitude of 1.0 G to 0.5 G gave high resolution as seen in black. Hyperfine coupling constants were then assigned to this highly resolved signal via the use of computer software. The simulation can be visualized in red just below the experimentally collected data. The determined hyperfine coupling constants of diAPAP were exactly half that of the APAP. The ortho protons, aH, and amide nitrogens, aN, had simulated constants of 2.53 G and 0.88 G compared to APAP’s simulated constants of 5.19 G and 1.68 G, respectively.
Given that, spiking the diAPAP sample with fractional APAP levels resulted in an increase in the radical concentration of diAPAP. The oxidation products associated with the solo diAPAP oxidation and the APAP spiked diAPAP oxidation through the IE-ESR system is portrayed in Figure 8. diAPAP oxidation resulting in the broadband ESR spectra of Figure 8 results in product formation of compound C, an acetaminophen tetramer. However, when spiked with fractional APAP, the main product outcome of diAPAP oxidation was compound B, an acetaminophen trimer, followed by compound C and further conjugated polymers.
Discussion
- The purpose of the discussion is to interpret the results and to relate them to existing knowledge in the field in as clear and brief a fashion as possible. Information given elsewhere in the manuscript should not be repeated in the discussion. Extensive reviews of the literature should be avoided.
Clearly, APAP oxidation is not so simple so as to only lead to the formation of one, clear cut, metabolite during oxidation in times of overdose. While NAPQI may be a product of APAP oxidation in the liver, products of a one electron oxidation cascade cannot be written out and remain prevalent. Five major APAP oxidation products were shown to be formed after modeling the CYP mediated reaction with HRP, a classic peroxidase. CYPs have been shown to catalyze one electron oxidations of phenolic compounds in environments of oxidative stress, which is certaintly the case with APAP overdose once glutathione levels have been depleted (4). Perhaps the metabolism of APAP after gluthione depletion favors the mechanism of radical polymerization as opposed to the net two electron oxidation of APAP into NAPQI. Considering the former could clear up discrepencies when considering the mystery of how APAP induced liver necrosis disseminates. For instance, while polyphenolic compounds are generally seen as safe supplements, high concentration seem to function antagonistically and were purported to lead to increases in DNA damage (12). If APAP is oxidized via a mechanism of one electron oxidation that yields polymers, then the concentration of polyphenols being formed would be classified as very high. This could elucidate the mechanism of mitochondrial dysfunction often associated with APAP overdose in the liver. If mitochondrial DNA is being damaged, then the kill switches, necrosis or apoptosis, could be signaled for in hepatocytes, thus bringing about APAP induced hepatotoxicity.
According to the ESR data collected, APAP appears to be most likely to react at the ortho carbon position to form an ortho-linked dimer (diAPAP). This is supported by previous APAP dimer identification performed by Potter in 1985; however, there was an additional geometric isomer of the APAP dimer that Potter did not characterize (11). The additional APAP dimer was found to be an ether-linked diAPAP molecule. Additionally, the diAPAP ESR indicates the mechanism of APAP oxidation by HRP is APAP mediated or APAP induced. In other words, APAP drives the reaction forward. To clarify, diAPAP does function as a substrate for the peroxidase, but does not yield large product fromation when compared to spiking the solution with low levels of APAP. Introducing APAP to the oxidation of diAPAP yielded far greater product outcomes as well as the depletion of diAPAP. It can be reasoned that APAP may be oxidized into a radical intermediate, which then directly adducts to diAPAP to form immediate APAP trimer or stimulates diAPAP oxidation by stealing an electron from diAPAP to generate the diAPAP radical and become reduced back to APAP.
References
1. Lee, W. M. (2013) Drug-induced acute liver failure. Clin Liver Dis. 17, 575-586.
2. Bernal, W., Lee, W. M., Wendon, J., Larsen, F. S., and Williams, R. (2015) Acute liver failure: A curable disease by 2024?. J. Hepatol. 62, 112-120.
3. Hinson, J. A., Reid, A. B., McCullough, S. S., and James, L. P. (2004) Acetaminophen‐Induced Hepatotoxicity:Role of Metabolic Activation, Reactive Oxygen/Nitrogen Species, and Mitochondrial Permeability Transition. Drug Metabolism Reviews 36(3-4), 805–822.
4. Potter, D. W. and Hinson, J. A. (1987) Mechanisms of acetaminophen oxidation to N-acetyl-P-benzoquinone imine by horseradish peroxidase and cytochrome P-450. J Biol Chem. 262, 966–973.
5. Gillette, J. R., Nelson, S. D., Mulder, G. J., Jollow, D. J., Mitchell, J. R., Pohl, L. R., and Hinson, J. A. (1981) Formation of chemically reactive metabolites of phenacetin and acetaminophen. Adv Exp Med Biol. 136, 931–950.
6. Dahlin, D. C., Miwa, G. T., Lu, A. Y., and Nelson, S. D. (1984) N-acetyl-p-benzoquinone imine: a cytochrome P-450-mediated oxidation product of acetaminophen. Proc. Natl. Acad. Sci. U. S. A. 81, 1327-1331.
7. Mitchell, J. R., Jollow, D. J., Potter, W. Z., Gillette, J. R., and Brodie, B. B. (1973b) Acetaminophen-induced hepatic necrosis. IV. Protective role of glutathione. J Pharmacol Exp Ther. 187, 211–217.
8. Ross, D., Norbeck, K., and Moldeus, P. (1985) The Generation and Subsequent Fate of Glutathionyl Radicals in Biological Systems. Journal of Biological Chemistry 260(28), 15028-15032.
9. West, P. R., Harman, L. S., Josephy, P. D., and Mason, R. P.(1984) Acetaminophen: enzymatic formation of a transient phenoxyl free radical. Journal of Biochemical Pharmacology 33(18), 2933-2936.
10. Fischer, V., West, P. R., Harman, L. S., and Mason, R. P. (1985) Free-Radical Metabolites of Acetaminophen and a Dimethylated Derivative. Environmental Health Perspectives64, 127-137.
11. Potter, D. W., Miller, D. W., and Hinson, J. A. (1985) Identification of Acetaminophen Polymerization Products Catalyzed by Horseradish Peroxidase. J. Biol. Chem. 260(12), 174-180.
12. Aqueta, A. and Collins, A. (2016) Polyphenols and DNA Damage: A Mixed Blessing. Nutrients 8(785), 1-21.
Funding Information
- Authors are required to report ALL funding sources and grant/award numbers relevant to this manuscript. Enter all sources of funding for ALL authors relevant to this manuscript in BOTH the Open Funder Registry tool in ACS Paragon Plus and in the manuscript to meet this requirement. See http://pubs.acs.org/page/4authors/funder_options.html for complete instructions.
Acknowledgment
- This section should acknowledge technical assistance, advice from colleagues, gifts, etc. Permission should be sought from persons whose contribution to the work is acknowledged in the manuscript.
Abbreviations
- Abbreviations are used in ACS Journals without periods. Standard abbreviations should be used throughout the manuscript. All nonstandard abbreviations should be kept to a minimum and must be defined in the text following their first use.
Footnotes
- When footnotes are necessary to express some relevant thoughts, these should be included as a parenthetical statement, placed next to the related text to ensure visibility. Footnotes should not be included in the reference list.
Tables
- Tabulation of experimental results is encouraged when this leads to more effective presentation or to more economical use of space. Tables may be created using a word processor’s text mode or table format feature. The table format feature is preferred. Ensure each data entry is in its own cell; no listing of data by using bullets or numbering. If the text mode is used, separate columns with a single tab and use a line feed (return) at the end of each row. Tables should be numbered consecutively with Arabic numerals. Provide a brief title with each table and a brief heading for each column. Clearly indicate the units of measure (preferably SI). Data should be rounded to the nearest significant figure. Explanatory material referring to the whole table is to be included as a footnote to the title (a). Footnotes in tables should be given lower case letter designations and cited in the tables as italicized superscripts. All tables should be cited in the text in consecutive order.
Previously published tables that are being borrowed or adapted from another source require permission from the copyright holder. Once permission is obtained, the permission letter should be uploaded to the submission under the tag “Other Files for Editors Only.” Also, the copyright holder’s preferred credit line should be included in the table’s legend.
Figures and Figure Legends. Line drawings, graphs, stereograms, histograms, and black and white (or color) photographs are all classified as figures and should be numbered consecutively with Arabic numerals in order of citation. Figure legends should be placed after the tables as a single list with the figures following. The figures must be embedded in the same document as the article and not submitted separately as Supporting Information. It may help to print the manuscript on a laser printer to ensure all artwork is clear and legible. See below for guidelines for preparing publication quality illustrations.
- Authors Photos and Biographies. All authors of Reviews and Perspectives (but not other publication formats) should provide a short biography (100 words or less) to be published with the articles.
Headshots (i.e., photos) of the authors may also be provided for publication with the biography. Photos and accompanying biography should be inserted below the reference list in the manuscript. Each photo must be high resolution (at least 300 ppi). No logos may appear in the photo. Before publication, each author must sign (1) a Model Release Form and (2) either a Copyright Transfer Form, which transfers copyright to ACS, or the Nonexclusive Rights Agreement, by which the copyright holder keeps the copyright but grants ACS permission to use the photo. Please choose either the Copyright Transfer Form or the Nonexclusive Rights Agreement; do not sign both forms.